Of late there seems to be an uptick in attention being paid to 6G. However, this is only the beginning of a movement, so there is little actual data in these reports. As a potential set of candidate frequencies for Beyond-5G or 6G, Terahertz (THz) frequencies are also receiving some attention. There are cursory discussions about what the THz bands could enable beyond 5G, where the research is, standards development, and such.
Little is mentioned about how deployment will work in practice, although there are technical papers from places like NYU, which has one of the best THz research programs in the world.
Forestalling THz Hype
The discussion should actually be framed as two separate topics: THz frequencies at or above 1 THz, and the 300 GHz to 1 THz (sub-THz or sub-mm) spectrum. The over-1 THz spectrum, where we have little data on how to implement wireless communications, is the most interesting but challenging. The sub-THz spectrum is much better studied and much of what happens up to about 150 GHz is well understood. As we go beyond that, particularly beyond 300 GHz, the problems become greater.
I touch on this because we should learn from the marketing hype that surrounded 5G. For discussions in THz to have real meaning, it is imperative we define the regions we are talking about by their scientific reference and not let marketers define it this time.
- Nearly all of the current discussions focus on sub-300 GHz frequencies, where wavelengths are one mm or longer. To a purist, any discussion below 300 GHz should remain in the mmWave camp.
- Anything above 300 GHz is less than 1mm long. Therefore, the term sub-THz or sub-mm can reference the frequencies between 300 GHz and 1 THz.
- Actual THz discussions should refer to frequencies at 1 THz or above.
The rest of this paper will focus on the real THz region, known as the “Terahertz gap” or far infrared. This is where the greatest challenges lie, particularly with radiating elements.
But before we start, perhaps a basic discussion on THz wave properties is in order.
The Physics of THz
One of the major issues 5G has faced is in managing radio propagation, particularly in the 60 GHz and higher spectrum. Radio engineers are all familiar with the fact that the higher in frequency one goes, the more the signal is affected by the environment (man-made and natural) and the less far it travels for a given power metric.
At frequencies between two and six GHz, the effect is minimal. Problems with propagation increase but not significantly and we have the technology to overcome them.
Above 6 GHz, things start to get problematic. Once frequencies go beyond 40 GHz things take a dramatic turn. Figure 1 details the effect of oxygen absorption across the mmWave spectrum. Figure 2 peeks at another issue, materials, and their effect on propagation.
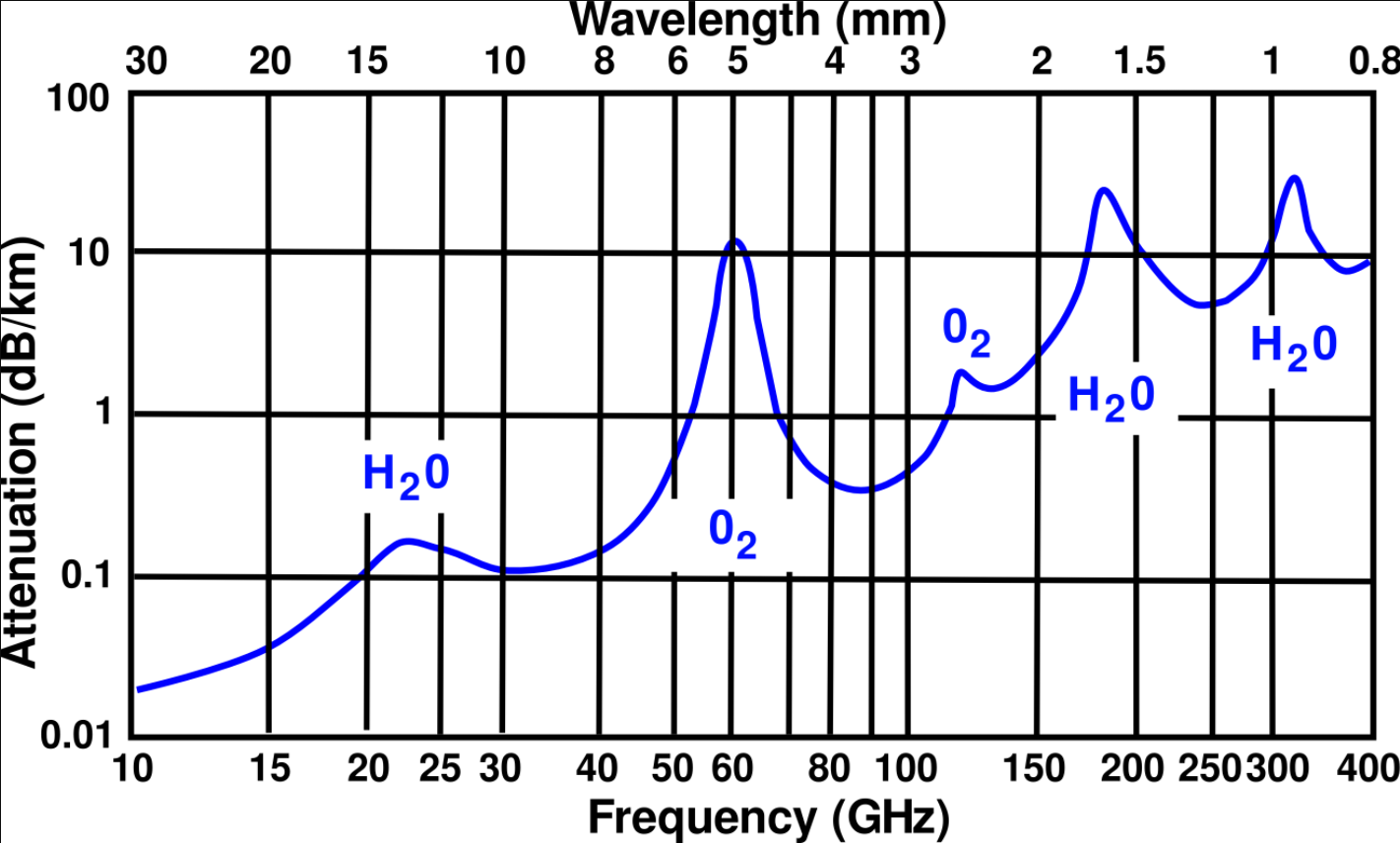
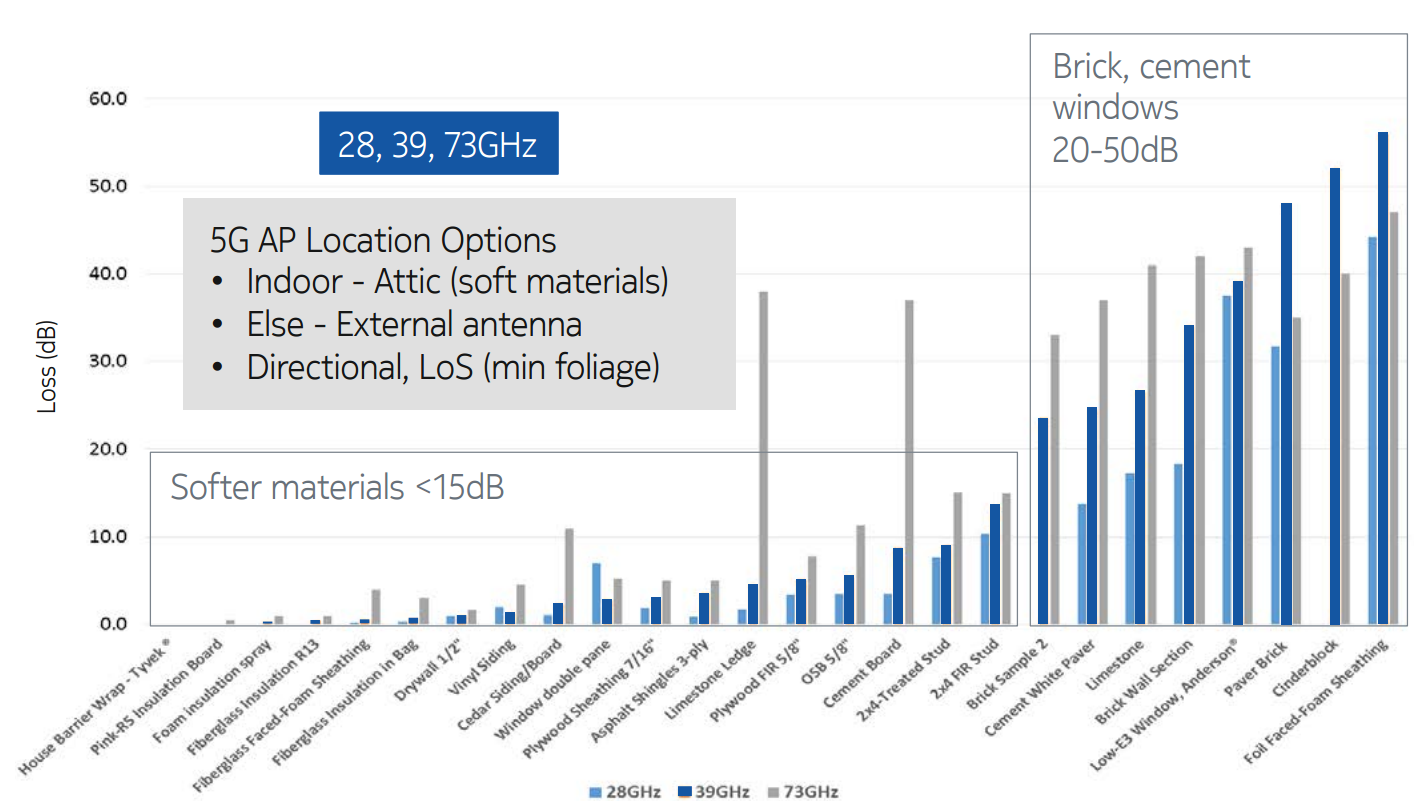
There are other issues, but the two charts visualize some of the more well-known issues. And, while we certainly have wireless communication in these frequencies, they are specialised and serve specific purposes.
Then we move on to the real challenges.
As we enter the Terahertz gap the wavelength of a 1THz signal is 300 µm or less. The propagation characteristics of these frequencies are orders of magnitude less robust than a 100 Ghz signal, the currently defined top of the 5G mmWave segment.
100 GHz signals are sensitive. It’s almost as though if you breathe in the signal path, it affects performance. If you increase that sensitivity by an order of magnitude for every additional 100 GHz then, by the time you get to the Terahertz gap, challenges abound.
One major challenge is power and efficiency. Another is the lack of devices with small enough band-gaps to work at these frequencies. Yet another is that today’s solid-state devices have the wrong physical properties to work effectively at such frequencies.
On top of that there is atmospheric absorption. This is seen in mmWave frequencies up to 300 GHz as well, but it is appreciably higher in the THz range (see Figure 3). The attenuation at 1 THz is over 1000 times that of the highest mmWave frequency.
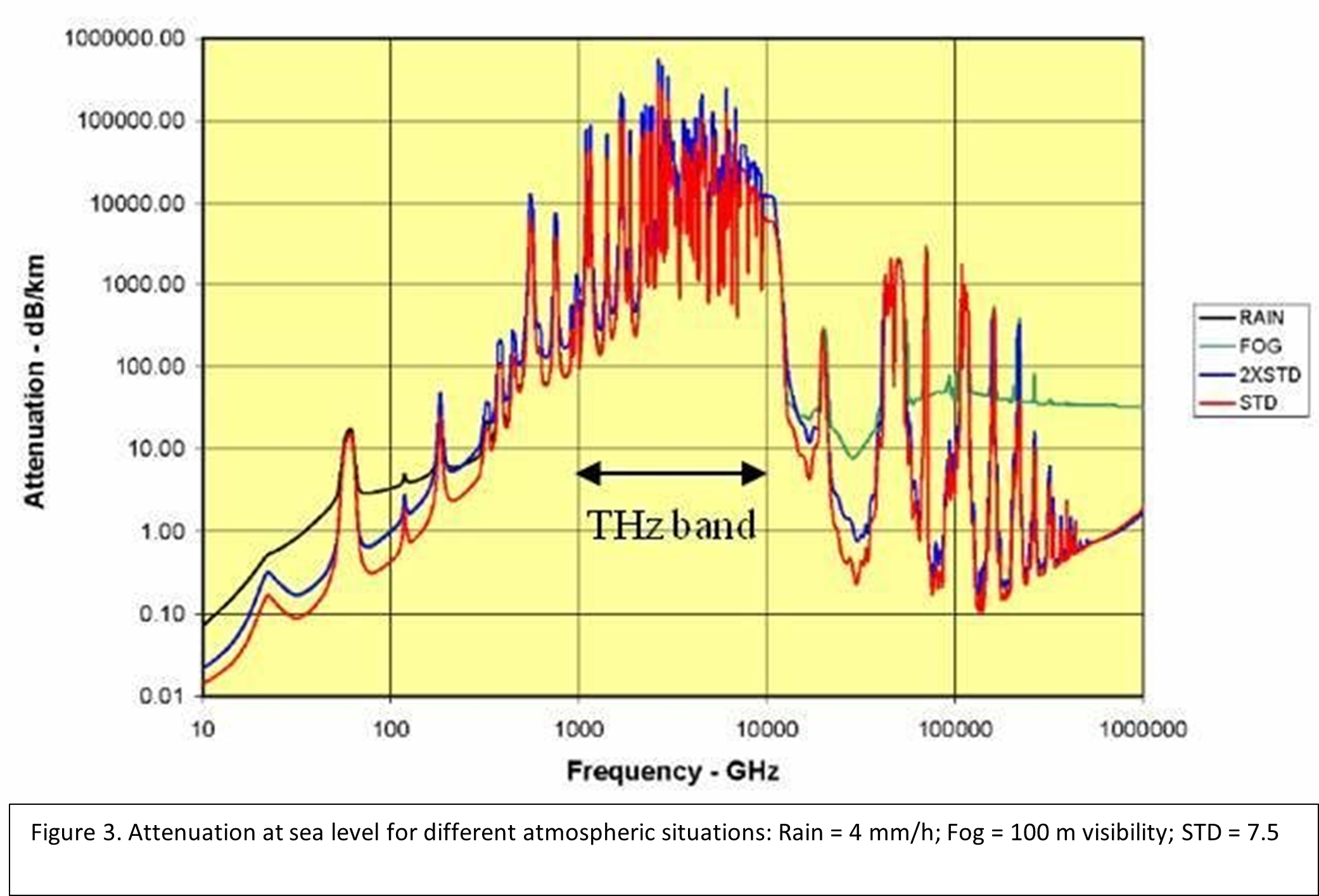
So, what does all this mean? Quite simply, THz communications will only be possible under very well controlled conditions – based on today’s knowledge, essentially very short-range communications. So far applications are being envisioned in medical imaging; personal security (body scanning); hazardous (explosive, chemical) material scanning; perhaps mail and small package screening. It is also being considered for semiconductor packaging inspections. In other words, very short distances.
In the longer term, forward thinkers are examining the use of THz spectrum for things like advanced holograms and massive-scale digital twin platforms. However, these will be much more challenging for signal transmission than scanning applications.
I mention these because they are good examples of how short the travel distance is for THz waves. Of course, this is all relative to power. However, scaling up power is not linear and introduces other issues.
Power Games and the Rise of RIS
For example, if one emits a 1-watt signal at a frequency of 1 THz, by the time it travels one km, it will have a signal strength of about 10-30 percent of its original strength. Even scaling up to a petawatt of power – which would be difficult without ionizing the atmosphere in the process – a receiver one km away would only receive a few femtowatts. Moreover, high-power THz waves have the same heating properties as sub-THz mmWave. The challenges of propagation over long distances are horrific.
As a result, for most applications THz frequencies will be limited to a few centimeters. Some tests have shown practical communications at a few meters, but that increases challenges exponentially so that will probably only occur in specialized applications.
Now let’s discuss the radiating elements for THz frequencies. One thing guaranteed is that radiating elements for THz will not resemble conventional antennae in most cases.
Since the length of a 1-THz signal is slightly less than 300 µm (about three times the thickness of average human hair), the only way to deliver coverage with conventional antennae would be to have billions of very small-scale micro base-stations everywhere – not realistic. A better solution is to turn surfaces into radiating elements or signal conductive paths.
This theory is not brand new. RF-friendly paint has been around for a few years now. Conductive cloths are another area of discussion; as are plastics, wood… just about any surface can, in theory, be turned into an RF radiator.
This would be an elegant solution to propagating THz waves and likely the only way ubiquity can be achieved. At the extreme, we could see a world where nearly every surface can be a THz radiator and signals simply hop and travel along from one surface to another. In practice there would be one big, connected network of hundreds of billions of devices and surfaces which, in an urban environment, humans have control over.
Now, let us not forget about more traditional antennas. The latest research is in THz photoconductive antennas, THz horn antennas, THz lens antennas, THz microstrip antennas, and THz on-chip antennas. However, these are currently only useful in sub-THz frequencies (up to 0.3 THz).
Eventually, sub-millimeter communications will be as common as positive ion drives for spacecraft. In time, all of this will move from the sci-fi camp to the can-do camp – just do not hold your breath.